Locating considerations of movement in design
For most of this book, movement in architectural design has been considered from the viewpoint of the observer/occupant.1 It is they who are expected to move through the synthesised environments contrived by the architect. Spatial devices such as the route, arcade, corridor, ramp, stair, pier, etc., attest to this. They are generally conceived as static elements, charged with the goal of steering occupant movement and framing socio-spatial practices such as the pilgrimage, the procession, the promenade or the dérive. We might call this approach to the consideration of movement – where the responsibility of movement is attributed to the observer – as anthropocentric.
There are notable works that experiment with a different point of departure in which movement is considered as a capacity of the proposition itself. For example, Ron Herron’s ‘A walking city’ (1964) conveys this ambition in the title alone.2 In this visionary proposition and its subsequent iterations, the city becomes itinerant, emancipating itself from geograph -ical fixity. The Maison du Peuple (1935) in Clichy, France, by Lods, Beaudouin, Prouvé and Bodiansky, was a built example, with movable floors: the building fabric was actively transformable to support multiple programmes, in the same location, at different times of day – a densification strategy that establishes synergies between programme, temporality and place. A third example is Brunelleschi’s device for testing the validity of his perspectival representa -tion of the Florentine Baptistry. It incorporated areas of burnished silver on the picture plane that directly reflected portions of the Baptistry’s context, constructing ‘live’ areas of movement within the representation.3
I suggest that the correspondence binding these three examples is a non-anthropocentric concern with movement – that is to say, the three architectural artefacts can be understood as con -cerned with movement arising from sources other than the observer. These three exemplify, respectively, the visionary, the built and the representational – realms necessarily interdepend -ent if one is engaging in the design and realisation of materially dynamic architectures. The experience of our research points to a need to develop synergetic relations between new conceptualisations, new methods of designing, new representational tools and new material systems. This chapter examines these issues through two interrelated research projects developed at the Centre for Information Technology and Architecture (CITA) as part of a broader enquiry at the intersection between architecture (considered as both practice and spatial construct) and digital technologies.
The Persistent Model project
The Persistent Model project examines how digital technologies can support new relations between representation and built artefact. The parent project is developed through discrete sub-projects. Each sub-project investigates specific questions by engagement with the development of new design tools and production of full-scale demonstrations, two of which have been completed and exhibited, and a third was under development at the time of writing. The origins of the overarching hypothesis for the project can be located in Stephen Groák’s assertion that, ‘The very conventions of representation also can affect the character of the buildings to which they subsequently give rise’.4 It follows that, if we reconstruct our methods of representation, we also construct the opportunity for realising qualitatively different spaces and places. The project engages three principal areas in which changes to the conventions of representation are considered: the duration of active influence that representation can hold in relation to the represented; the means, methods and media through which representations are constructed and used; and what it is that is being represented. These concerns with representation are tuned towards operating with material dynamics as a principal design driver and their deployment in dynamic architectures – material assemblies with an inherent capacity for movement.
Free-form metal inflation is a fabrication process that establishes a dependence between the given boundary profile of a ‘cushion’ component and the material mechanisms at work when the steel cushion moves, under internal loading, from the elastic to the plastic regime. This is where introduced energy starts to become irrecoverable, and permanent forming occurs. As the component is a cushion, the act of inflation increases the cross-sectional area, but, as the pressures involved are low, there is negligible change in the total surface area. Depending on the boundary profile, this leads to self-generating buckling phenomena and a material reluctance to form double curvature. Another point of interest is that the inflation process can be arrested and resumed arbitrarily, allowing components to be partially inflated.5 This segues to the interesting notion of ‘harvesting’ the performance potential of any individual component, as required over time.6 The two projects described below took free-form metal inflation as a starting point for architectural constructs with an inherent potential capacity for movement.
Persistent Model #1
The first full-scale demonstration – Persistent Model #1 – was developed for the CITA exhibition digital.material, held at the ROM Gallery for Art and Architecture, Oslo, in 2010. The exhibition comprised four CITA projects, each offering nuanced perspectives on digital design practice and its role in enabling a new culture of material thinking in architectural design. The four full-scale demonstrations – Reef Pattern, Thaw, Lamella and Persistent Model #1 – showed how digital tools can enable extensions to existing material and craft practices and their associated logics of construction. Particular attention was given to designing with material performance as a design driver, and various trajectories of investigation were explored, based on this common starting point.7 Persistent Model #1 explored how digital technologies permit the reconsideration of relationships between representation and realisation. We wanted to know how digital technologies might support continuous feedback between spaces of representation and realisation, and what material system could allow driveable spatial transforms over time. A feedback model would support the ability to incorporate unanticipated transforms in the material system and open the way for further design speculation. Steel cushions formed through low-pressure inflation allowed us to investigate significant spatial movements. These movements started as mechanical transforms at the material level and then percolated through component and assembly to organisations of material and component. Inflation of the assembly began at the opening of the exhibition to dramatise the dynamic process.
A significant design challenge was the fact that the inflation process caused the component to deviate from the initial drawings used to cut the profiles (Figure 4.7.1), thereby making preceding representations redundant. The representational question arose of how these transformations in component attributes could be captured as operational and explicit parameters in a design context. In Persistent Model #1, this question was compounded by the fact that the assembly’s constituent components were selectively inflated, causing transforms across hierarchies of scale (from material to component to assembly) and defying our ability to model the result predictively by digital means.
A representational model was established alongside the built construct, introducing a feedback loop between the space of design and the space of material presence (Figure 4.7.2). Changes to the physical construct were mapped back on to an abstracted representation, allowing subsequent decisions to be made on the basis of the as-built condition, rather than an idealised condition. The role of the model, therefore, shifted between recording the actual changing conditions and providing the basis for subsequent speculation and specification. The resulting digital construct possessed the qualities of being steered yet unanticipated, both deliberate and tempered by dynamic behaviour of the material (Figure 4.7.3).
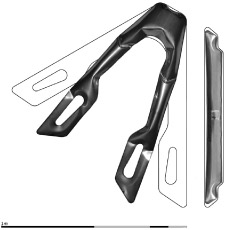
Figure 4.7.1 The initial profile geometry of free-form inflated metal cushions is a primary determinant of the extent of deviation exhibited under inflation
Source: Phil Ayres/CITA
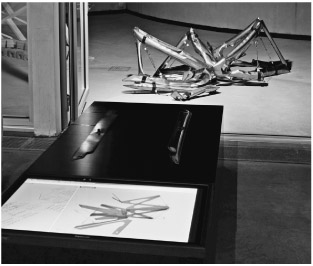
Figure 4.7.2 Persistent Model #1 presented the digital model and the physical artefact as a linked system in which physical movement under inflation could be mapped back to the space of representation
Source: Anders Ingvarsten
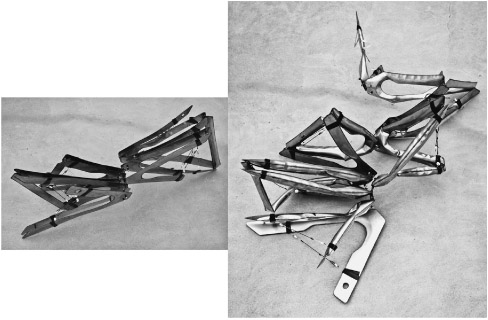
Figure 4.7.3 The arrangement of the physical assembly at the start of the exhibition is shown on the left, and the arrangement by the end of the exhibition is on the right. The spatial movements resulted from the careful junctioning of components to ‘amplify’ the material dynamics resulting from inflation
Source: Anders Ingvarsten
Persistent Model #2
The opportunity to develop a second project arose out of a successful funding application and the support of the ggggallery™, Copenhagen, which ran an innovative programme of architecture exhibitions at the intersection of experiment and technology. The gallery’s programme, which ran for more than 2 years, focused on architectural research enquiry in which the making of physical artefacts, prototypes and demonstrations was central. Persistent Model #2: dynamic pressure systems opened in February 2013 and ran until July 2013. The exhibition comprised a single, full-scale tensegrity system, approximately 5 m long, 3 m high and 1.5 m deep, like a wall along one side of the gallery. Persistent Model #2 took as its point of departure a more appropriate tectonic logic for inflatable metal cushions. It followed the simple principle that, if the metal cushions were to maintain the capacity to transform though repeated inflations and deflations, the integrity of the membrane needed to be preserved. This led to an avoidance of junctions and metal fixings that puncture the material (bolting, riveting, screwing). We also wanted to expand the vocabulary of inflatables, complementing the hard, irreversible transformations of the metal cushions with soft, reversible ones.
Tensegrity structures possess a bounding net of continuous tension in which compressive members are held without touching one another, the whole achieving a state of equilibrium. The tectonic implication is, therefore, that junctions between compression members can be dispensed with. However, this depends how the notion of compression members is defined in relation to specific tensegrity topologies. The planar double-layer topology of Persistent Model #2, adopted from Kenneth Snelson’s Planar Weave of 1960,8 exhibits clear groupings of linked compression members (Figure 4.7.4). However, René Motro defines the notion of ‘set’, and, crucially, discontinuity between sets, as a means of accommodating this type of configuration within the definition of tensegrity systems.9 From a tectonic perspective, this required that we devise a novel means of connecting cushions while preserving their integrity. This was achieved by employing a material dynamics mechanism steered through defined geometry that we had discovered in the earlier project (see Figure 4.7.1). A ‘chevron’ geometry, applied at a reduced scale to the ends of linear bar components, allowed clamping on to a flat steel junction by a process of inflation. This flat, wing-like component also incorporated the junction of the tensile members at geometric node points (Figure 4.7.5). Such tensegrity structures can be considered analogous to inflatable membranes. The analogy holds if a balloon is considered as comprising two components: a bounding envelope that exhibits tensile con -tinuity and the contained air that acts as the compressed component (Motro, 2003).10
Fuller is also clear about this conceptualisation, stating that, ‘Tensegrity structures are pure pneumatic structures and can accomplish visibly differentiated tension-compression interfunc -tioning in the same manner that is accomplished by pneumatic structures, at the subvisible level of energy events’.11
The conceptual congruence between tensegrities and inflatables afforded an understanding that multi-scalar dependencies and relations of similarity could be established between the parts and whole of a materially variegated system – a system of nested tensegrities composed of actively inflated and elastic membranes (foils) and plastically formed membranes with potential for further inflation (steel cushions).
In this project, the characteristics of Snelson’s Planar Weave were reconsidered from an architectural sensibility, seeking synergies between structural logic, component demands and material dynamics. Further concerns were issues of skin, local specificity and production of
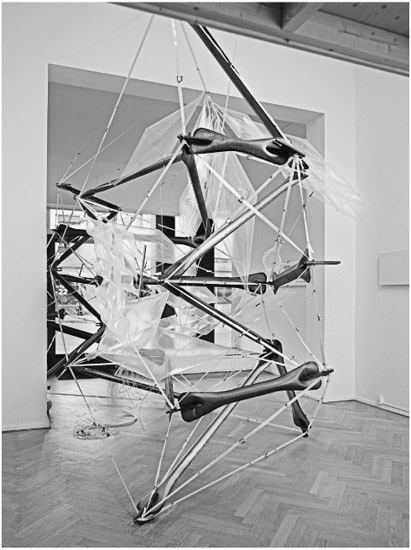
Figure 4.7.4 Persistent Model #2 began as an exploration of a more appropriate tectonic for the inflatable metal cushions, in order to preserve their integrity as an inflatable body. The resulting structure was an irregular, double-layer tensegrity
Source: Anders Ingvarsten
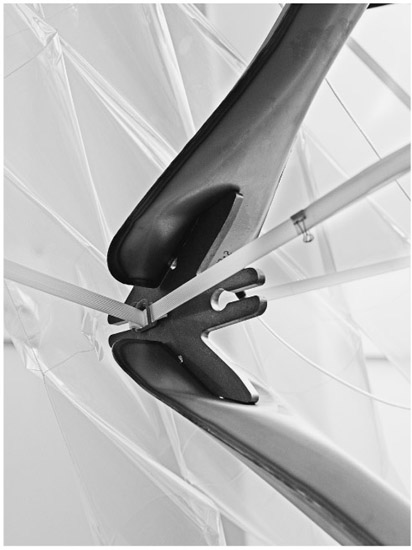
Figure 4.7.5 A tensegrity node point with ‘soft’ inflatable skin applied. Nodes are the locations where compression and tension members meet
Source: Anders Ingvarsten
variety through a geometrically irregular expression of the topology. These reconsiderations were examined and supported through a bespoke computational infrastructure with different concerns to those of Persistent Model #1. Where previously the digital infrastructure was tuned to support feedback between the space of representation and the changing states of the physical construct, with Persistent Model #2, the digital infrastructure was tuned towards the exploration, simulation and performance analysis of the geometries achieved with the chosen tensegrity topology. The investigation of irregular geometries required a method of simulation to ensure that the tensegrity could achieve a state of structural stability, or equilibrium. The digital infrastructure also incorporated the ability to generate fabrication data in real time, for the production of scale models or full-scale prototypes (Figure 4.7.6). This link back into physical modelling and prototyping provided a mode of testing through which to calibrate and tune the material dynamics encoded in the digital infrastructure.
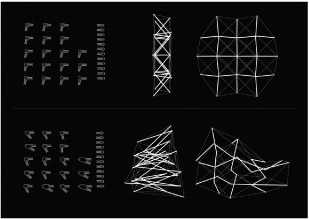
Figure 4.7.6 The digital infrastructure developed for the project allowed real-time exploration of variant geometries and supported the generation of fabrication data, in this case defining elements for the production of scale physical models
Source: Phil Ayres/CITA
Although Persistent Model #2 was not designed for active movement, it was nevertheless a dynamic and ‘live’ tensile structure that reconfigured itself to applied loads. As we have developed a proof-of-concept for both the digital infrastructure as design/analysis tool and the material system, future work will focus more directly on controlling shape change through inherent mechanisms of material movement – both elastic (temporary) and plastic (permanent).
Conclusion
The two projects described above consider some challenges and opportunities of design practices and the resulting constructs when material dynamics is taken as the main design driver. Enlisting mechanical material behaviours to induce non-anthropocentric spatial movement requires design tools that support an investigation of scalar dependencies. It requires the ability to extrapolate the dynamic spatial and performance implications of differing material organisations. It requires the development of novel tectonic systems, tuned to operate sensitively with dynamics occurring at the material level. It may even require the reconsideration of conventional relations between representation and artefact.
The Persistent Model project shows the possibility of models intended to be incomplete, open ended and attuned to being informed by the complexities and dynamics of the material world. Although the term persistent model could be interpreted as an aspiration towards seamless model integration, our experience of the two projects has caused us to look critically upon this aim. Our practice is not so much about establishing seamless integration between all aspects of the design environment, as about understanding and articulating the inter -dependencies between modes of modelling – digital and analogue. This suggests establishing an ‘ecology-of-models’, rather than a single, integrated model. Such an ecology would seek productiveness, not only in the correspondences, but also in the gaps and differences, and would certainly offer a clearer strategy for the inclusion of the role that physical models and 1:1 prototypes play. For, in this, and many other CITA projects, the aim has not just been instrumental, but has been a critical starting point through which to inform and calibrate an encoding of material dynamics within our design environments.
The project demonstrates a design-led probing of the relationship between representation and the represented. The notion of persistent modelling might be understood as a familiar activity to architectural design if considered as describing an active and iterative engagement with design concerns. However, the persistence in persistent modelling can also be understood to apply in other ways, reflecting and anticipating roles for representation that could support architectures of persistent transformation and movement.
Acknowledgements
Persistent Model #1 was funded by CITA and received industry support from Beyer Teknik. The design team was Phil Ayres and Anders Holden Deleuran.
Persistent Model #2 was funded by DreyersFond and the Royal Danish Academy of Fine Arts, School of Architecture. It received industry support from Beyer Teknik, Amcor and Festo.
The design team was Phil Ayres, Anders Krogdal Nielsen, Kristjana Sigurdardottir and Roxana Aron.
Notes
1 The notion and scale of movement referred to here are above those concerned with technical detailing.
2 See R. Herron (1964) A walking city, Archigram, vol. 5, p. 17.
3 It is the device itself that is of interest here, rather than the perspectival representation of the Baptistry that Brunelleschi was testing through this viewing tool. The device was a sophisticated construct in which the observer looked from the back of the picture plane, through a hole, to a mirror that reflected the content of the picture plane. The viewing procedure required the observer to be precisely located in front, but looking away from the Baptistry doors. The viewing hole in the picture plane corresponded to the eye height of the observer in ‘real space’. The effect would, therefore, be a representational equivalent of viewing the Baptistry from a point out in the surrounding square, with live movement incorporated into the upper levels of the scene by virtue of the burnished silver areas on the picture plane. Eugenio Battisti provides a thorough analysis of the device, based on a reconstruction and use (Battisti 2002).
4 Groák 1992.
5 See P. Ayres ‘Microstructure, macrostructure and the steering of material proclivities’, in Sheil 2012, pp. 220–37.
6 The notion of ‘harvesting’ performance describes the ability to arbitrarily increase the performance capacity of components if they are not inflated to their material limits. Performance capacity here refers to an increase in bending resistance through an increase in cross-sectional area of the component. The action of ‘harvesting’ would, therefore, assume an initial redundancy – prior inflations remaining below the material limits.
7 See Ayres et al. 2012, and P. Ayres, M. Tamke and M. Ramsgard Thomsen, ‘Making a digital-material practice’ in Ng and Patel 2013, pp.125–44.
8 See K. Snelson (2012) The art of tensegrity, International Journal of Space Structures, vol.27, no. 2/3, pp. 71–80.
9 Motro 2003.
10 Ibid.
11 Fuller 1975.